
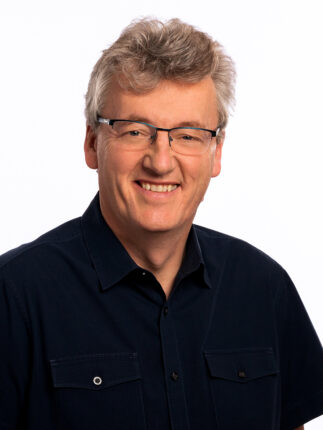
David MacMillan
Contact:
David MacMillan
James S. McDonnell Distinguished University, Professor of Chemistry
[email protected]
Frick Laboratory, 192
609-258-3916
Faculty Assistant:
Caroline Phillips
[email protected]
Frick Laboratory, 191
609-258-2254
Research Focus
Research in the MacMillan Group is focused on organic synthesis, catalysis, and chemical biology. We aim to pursue new concepts in synthetic organic chemistry that allow access to structural motifs not readily available via conventional methods. In this endeavor, we target the development of general strategies—organocatalysis, cascade, synergistic, photoredox, and metallaphotoredox catalysis—that can be implemented in a wide spectrum of chemical processes, and we place particular emphasis on the synthesis of moieties prevalent in medicinal agents, agrochemicals, and natural products. Our emerging chemical biology research program leverages photocatalysis to investigate complex biological environments.
Areas of active research include:
Photoredox catalysis. Since its early beginnings, the field of photoredox catalysis has undergone substantial expansion. Today, photoredox catalysis serves as a focus of research for more than 300 academic groups, and thousands of manuscripts have been published in this area. This notable growth can be attributed to the fact that photoredox: (1) selectively delivers ~60 kcal/mol of energy in the form of visible light to a photocatalyst, enabling the generation of highly reactive free radicals at room temperature and resulting in exquisite functional group tolerance; (2) utilizes feedstock chemical functionalities such as alcohols, olefins, carboxylic acids, and C-H bonds as radical precursors; and (3) has a mild, yet robust nature, allowing for merger with other catalytic approaches, including organocatalysis, NHC, hydrogen-atom-transfer (HAT), Lewis acids, and transition-metal catalysis.
Our laboratory has played a part in the development of photoredox catalysis over the last 17 years, with more than 100 published works, of which more than 20 have been featured in Science or Nature. We aspire to continue to grow the chemist’s synthetic toolbox of transformations that are novel yet highly adaptable across the many fields of science that rely upon molecule construction.
Metallaphotoredox catalysis. In a 2014 collaboration with the Doyle group, we reported one of the first examples of merging transition metal catalysis and photoredox catalysis to access novel reactivity that cannot be achieved by either catalytic system individually. Over the past decade, this new field of catalysis has delivered a host of highly enabling C–C and C–X bond-forming reactions that reveal previously elusive synthetic disconnections. Our group’s research in this area of metallaphotoredox catalysis is primarily focused on two metals: nickel and copper. By combining the fundamental mechanistic steps of nickel catalysis, such as oxidative addition and reductive elimination, with high-energy radicals generated through photoredox catalysis, we have been able to develop a broad range of novel C(sp2)–C(sp3) and C(sp3)–C(sp3) bond forming reactions. More recently, we demonstrated that copper catalysis could be successfully merged with photoredox catalysis, establishing a new catalytic platform with orthogonal reactivity to previous metallaphotoredox systems. The ability to access elusive high-energy copper intermediates under exceedingly mild conditions has unlocked new synthetic transformations, including C–CF3, C–X, C–N, and C–C bond formations. Our metallaphotoredox research program continues to focus on the development of new methods that accelerate the synthesis of complex organic molecules.
Radical sorting. In 2021, we disclosed one of the first applications of SH2 radical sorting to chemical methodology. Previously, the bimolecular homolytic substitution (SH2) mechanism had been well-known in biological systems but had not yet been harnessed as a general platform for cross-coupling reactions in the laboratory. We showed that this platform could be used to enable previously inaccessible disconnections. Traditional inner sphere bond-forming mechanisms proceed through the intermediacy of unstable dialkyl metal complexes that are prone to decomposition. By contrast, the innate outer sphere nature of bond formation via SH2 radical sorting circumvents this requirement, enabling the synthesis of historically challenging motifs such as all-aliphatic quaternary carbons. In principle, any two radical precursors might be used to form a new C–C bond within this paradigm, underscoring the immense synthetic potential for this platform. Indeed, the overarching goal for our radical sorting platform is to view any two functional groups as precursors to C(sp3)–C(sp3) bonds.
Chemical Biology. A current focus of the MacMillan group is the development and application of synthetic tools to address outstanding questions in chemical biology. In a 2020 Science paper, we introduced a new photocatalytic proximity labeling platform capable of elucidating interactions between proteins in biological systems with significantly enhanced spatial resolution compared to traditional methods. Application of this platform, which we term micromap (µ-map), has enabled us to make key discoveries in the areas of glycoprotein and chromatin biology. In 2023, we demonstrated that the exquisite special resolution inherent to µ-map allows for the identification of small molecule binding sites, circumventing the need for labor-intensive protein crystallization. We continue to refine and apply the µ-map technology with the goal of leveraging µ-map to investigate diverse facets of biology at the sub-cellular levels.
In our efforts to rapidly discover and optimize new reaction methodologies, we have capitalized on the capabilities of the Merck Center for Catalysis at Princeton University, which is a unique state-of-the-art facility that enables the high-throughput execution and analysis of catalytic reactions. The center houses a Chemspeed Accelerator robotic platform, an automated system used to apply the center’s resources to accelerate challenging, high-value projects for both methodology development and optimization of key steps in total synthesis.
Honors
Knight Bachelor (2022)
Nobel Prize in Chemistry (2021)
Centenary Prize, Royal Society of Chemistry, UK (2020)
Nagoya Medal, Nagoya University (2019)
Elected to the National Academy of Sciences, USA (2019)
ACS Gabor Somorjai Award in Catalysis (2018)
Noyori Prize, Japanese Society of Synthetic Chemistry (2018)
Henry J. Albert Award from BASF and IMPI (2017)
Janssen Pharmaceutical Prize, Belgium (2016)
Ohio State Edward Mack Jr. Award (2016)
Tischler Award, Harvard University, MA 2016 ACS Kosolapoff Award (2016)
Hamilton Award in Molecular Sciences, University of Nebraska (2015)
Schering Foundation Prize (Berlin) for Outstanding Research in Medicine, Biology or Chemistry (2015)
NJ ACS Award for Creativity in Molecular Design and Synthesis (2014)
Harrison Howe ACS Award in Chemistry (2013)
Elected to the Fellowship of the Royal Society (FRS, 2012)
Elected to the American Academy of Arts and Sciences (2012)
ACS Prize for Creative Work in Organic Synthesis (2011)
Mitsui Award in Catalysis (2011)
Mukaiyama Prize (Japanese Society of Organic Chemists, 2007)
Arthur C. Cope Scholar ACS Award (2007)
Thieme-IUPAC Prize in Synthetic Organic Chemistry (2006)
Elias J. Corey ACS Award (2005)
Selected Publications
“Exploiting the Marcus inverted region for first-row transition metal-based photoredox catalysis.” Chan, A. Y.; Ghosh, A.; Yarranton, J. T.; Twilton, J.; Jin, J.; Arias-Rotondo, D. M.; Sakai, H. A.; McCusker, J. K.; MacMillan, D. W. C. Science 2023, 382, 191.
“Tracking Chromatin State Changes Using Nanoscale Photo-Proximity Labelling.” Seath, C. P.; Burton, A. J.; Sun, X.; Lee, G.; Kleiner, R. E.; MacMillan, D. W. C.; Muir, T. W. Nature 2023, 616, 574.
“Rapid and Modular Access to Quaternary Carbons from Tertiary Alcohols via Bimolecular Homolytic Substitution.” Gould, C. A.; Pace, A. L.; MacMillan, D. W. C. Am. Chem. Soc. 2023, 145, 16330.
“General Access to Cubanes as Benzene Bioisosteres.” Wiesenfeldt, M. P.; Rossi-Ashton, J. A.; Perry, I. B.; Diesel, J.; Garry, O. L.; Bartels, F.; Coote, S. C.; Ma, X.; Yeung, C. S.; Bennett, D. J.; MacMillan, D. W. C. Nature 2023, 618, 513.
“µMap Photoproximity Labeling Enables Small Molecule Binding Site Mapping.” Huth, S. W.; Oakley, J. V.; Seath, C. P.; Geri, J. B.; Trowbridge, A. D.; Parker, D. L.; Rodriguez-Rivera, F. P.; Schwaid, A. G.; Ramil, C.; Ryu, K. A.; White, C. H.; Fadeyi, O. O.; Oslund, R. C.; MacMillan, D. W. C. Am. Chem. Soc. 2023, 145, 16289.
“Metallaphotoredox-enabled deoxygenative arylation of alcohols” Dong, Z.; D. W. C. MacMillan; Nature 2021, 598, 451.
“Microenvironment mapping via Dexter energy transfer on immune cells.” Geri, J. B.; Oakley, J. V.; Reyes-Robles, T; Wang, T.; McCarver, S.; White, C. H.; Rodriguez-Rivera, F. P.; Parker D. L. Jr.; Hett, E. C.; Fadeyi, O. O.; Oslund, R. C. W. C. MacMillan; Science 2020, 367, 1091.
“A Radical Approach to the Copper Oxidative Addition Problem: Trifluoromethylation of Bromoarenes.” Le, C.; Chen, T. Q.; Liang, T.; Zhang, P.; MacMillan, D. W. C. Science 2018, 360, 1010.
“Direct Arylation of Strong Aliphatic C–H Bonds.” Perry, I. B.; Brewer, T. F.; Sarver, P. J.; Schultz, D. M.; DiRocco, D. A.; MacMillan, D. W. C. Nature 2018, 560, 70.
“Photosensitized, energy transfer-mediated organometallic catalysis through electronically excited nickel(II).” Welin, E. R.; Le, C.; Lin, S.; Arias-Rotondo, D. M.; McCusker, J. K.; MacMillan, D. W. C. Science 2017, 355, 380.
“Selective sp3 C–H Alkylation via Polarity Match Based Cross-Coupling.” Le, C.; Liang, Y.; Evans, R. W.; Li, X.; MacMillan, D. W. C. Nature 2017, 547, 79.
“Native functionality in triple catalytic cross-coupling: sp3 C–H bonds as latent nucleophiles.” Shaw, M. S.; Shurtleff, V. W.; Terrett, J. A.; Cuthbertson, J. D.; MacMillan, D. W. C. Science 2016, 352, 1304.
“Aryl amination using ligand-free Ni(II) salts and photoredox catalysis.” Corcoran, E. B.; Pirnot, M. T.; Lin, S.; Dreher, S. D.; DiRocco, D. A.; Davies, I. W.; Buchwald, S. L.; MacMillan, D. W. C. Science 2016, 353, 279.
“Metallaphotoredox-catalyzed sp3–sp3 cross-coupling of carboxylic acids with alkyl halides” Johnson, C. P.; Smith, R. T.; Allmendinger, S.; MacMillan, D. W. C. Nature 2016, 536, 322.
“Switching on Elusive Organometallic Mechanisms with Photoredox Catalysis.” Terrett, J. A.; Cuthbertson, J. D.; Shurtleff, V. W.; MacMillan, D. W. C. Nature 2015, 524, 330.
“Alcohols as alkylating agents in heteroarene C–H functionalization.” Jin, J.; MacMillan, D. W. C. Nature 2015, 525, 87.
“Merging photoredox with nickel catalysis: Coupling of a-carboxyl sp3-carbons with aryl halides.” Zuo, Z.; Ahneman, D.; Chu, L.; Terrett, J.; Doyle, A. G.; MacMillan, D. W. C. Science 2014, 345, 437.
“Trifluoromethylation of Arenes and Heteroarenes via Photoredox Catalysis.” Nagib, D. A.; MacMillan, D. W. C. Nature 2011, 480, 224.
“Enantioselective a-Trifluoromethylation of Aldehydes via Photoredox Organocatalysis.” Nagib, D. A.; Scott, M. E.; MacMillan, D. W. C. Am. Chem. Soc. 2009, 131, 10875.
“Merging Photoredox Catalysis with Organocatalysis: The Direct Asymmetric Alkylation of Aldehydes.” Nicewicz, D. A.; MacMillan, D. W. C. Science 2008, 322, 77.
“Enantioselective Organocatalytic Hydride Reduction.” Ouellet, S. G.; Tuttle, J. B.; MacMillan, D. W. C. Am. Chem. Soc. 2005, 127, 32.
“Two-Step Synthesis of Carbohydrates by Selective Aldol Reactions.” Northrup, A. B.; MacMillan, D. W. C. Science 2004, 305, 1752.
“Enantioselective Organocatalytic Indole Alkylations. Design of a New and Highly Effective Chiral Amine for Iminium Catalysis.” Austin, J. A.; MacMillan, D. W. C. Am. Chem. Soc. 2002, 124, 1172.
“The First Direct and Enantioselective Cross-Aldol Reaction of Aldehydes.” Northrup, A. B.; MacMillan, D. W. C. Am. Chem. Soc. 2002, 124, 6798.
“New Strategies for Organic Catalysis: The First Highly Enantioselective Organocatalytic Diels–Alder Reaction.” Ahrendt, K. A.; Borths, C. J.; MacMillan, D. W. C. Am. Chem. Soc. 2000, 122, 4243.
Related News
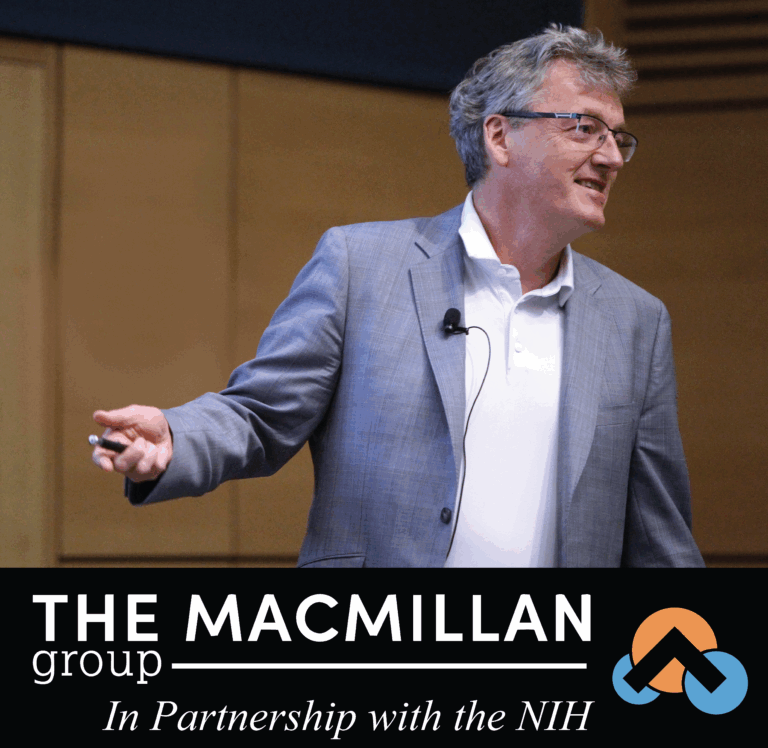
In partnership with the NIH: The David MacMillan Group and photoredox catalysis
MacMillan named Ludwig Distinguished Scholar; heralds new cancer research mission for lab
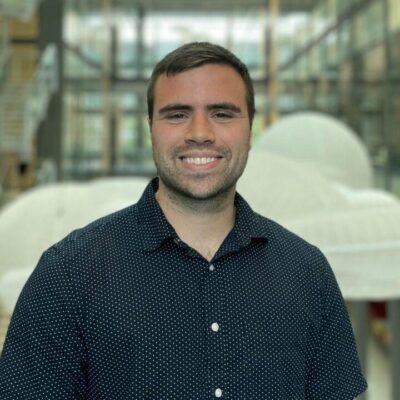