
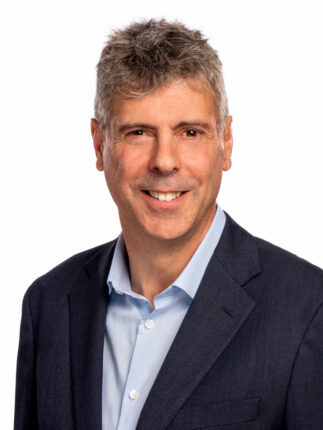
Gregory Scholes
Contact:
Gregory Scholes
William S. Tod Professor of Chemistry
[email protected]
Frick Laboratory, 125
609-258-0729
BioLEC Website
Faculty Assistant:
Nagwan I. Ali
Faculty / Grants Assistant
[email protected]
Frick Laboratory, 389
609-258-2330
Research Focus
The Scholes Group studies how complex molecular systems in chemistry and biology interact with light. We are interested to learn the mechanisms for photo-initiated processes like solar energy conversion. We are also working out how quantum-mechanical phenomena influence function. In our research we use ultrafast lasers to time processes and reveal unforeseen details using multidimensional electronic spectroscopy. Analysis and deeper understanding of our experiments is helped by quantum chemical calculations and by developing and applying theoretical models.
We study a broad range of projects. These include projects that address questions in quantum information science, photobiomodulation medicine, cavity quantum electrodynamics, photosynthesis, solar energy conversion, excitonic materials, and mechanisms of photo-activated catalysis in synthetic chemistry.
Insights into superfast molecular transformations and dynamics. A key consequence of impulsive broad-band laser excitation is that the ensemble of chromophores is synchronized. What this means is that we can probe properties intrinsic to the chromophores, which would otherwise be obscured by ensemble averaging. We perform such experiments using various coherence spectroscopies enabled by ultrafast laser science. In particular, we produce and analyze “wavepackets”.
In recent work, the Scholes group has used coherence spectroscopies to delve deeper into the origin and nature of coherence phenomena in complex systems, electron and proton transfer dynamics, transition metal complexes, and excitonic systems. We have also used coherence to learn about the mechanisms underpinning condensed phase dynamics in systems ranging from semiconductors to electron transfer reactions. Scholes led a recent Department of Energy workshop to discuss the future of the coherence field, which resulted in a viewpoint paper published in Naturein 2017. Current initiatives include discovering how the wave basis of quantum theory can be evidenced in a chemical reaction and how quantum correlations can be detected. Challenges include: Where do we look for these quantum effects, which are typically hidden or rapidly lost? What experiments might reveal them? A breakthrough in this area will enable ways of encoding, manipulating, and using quantum information in chemical systems.
Broad-band femtosecond spectroscopy choreographs ensembles. Time-dependent quantum mechanics provides a holistic description of a molecule’s structure and dynamics over all degrees of freedom, and can generally be related back to our familiar time-independent view by a simple Fourier transform. In the same way, time-resolved coherent spectroscopy is a comprehensive method for spectroscopy and dynamics. It provides time-domain information in the form of photo-initiated dynamics with a resolution down to femtoseconds, while simultaneously revealing a molecule’s frequency-domain spectroscopic content at a level of detail rarely realized by steady-state methodologies. The latter feature comes in the form of coherences, or wavepackets, whose time evolution maps out the complex multidimensional potential energy surfaces of the molecule.
Read more:
(1) Gregory D. Scholes, Graham R. Fleming, Lin X. Chen, Alán Aspuru-Guzik, Andreas Buchleitner, David F. Coker, Gregory S. Engel, Rienk van Grondelle, Akihito Ishizaki, David M. Jonas, Jeff S. Lundeen, James K. McCusker, Shaul Mukamel, Jennifer P. Ogilvie, Alexandra Olaya-Castro, Mark A. Ratner, Frank C. Spano, K. Birgitta Whaley, Xiaoyang Zhu “Utilizing Coherence to Enhance Function in Chemical and Biophysical Systems” Nature 2017, 543, 647–656.
(2) Shahnawaz Rafiq, Bo Fu, Bryan Kudisch, and Gregory D. Scholes. “Interplay of vibrational wavepackets during an ultrafast electron transfer reaction” Nat. Chem. 2021, 13, 70–76.
(3) Shahnawaz Rafiq and Gregory D. Scholes, “From Fundamental Theories to Quantum Coherences in Electron Transfer” J. Am. Chem. Soc. 2019, 141, 708–722.
(4) Kyra N. Schwarz, Paul B. Geraghty, Valerie D. Mitchell, Saeed-Uz-Zaman Khan, Bryan Kudisch, Jegadesan Subbiah, Barry P. Rand, Trevor A. Smith, Gregory D. Scholes, David J. Jones, Kenneth P. Ghiggino ” Reduced Recombination and Capacitor-like Charge Buildup in an Organic Heterojunction” J. Am. Chem. Soc. 2020, 142, 2562-2571.
(5) Shahnawaz Rafiq, Maté J. Bezdek, Paul J. Chirik, and Gregory D. Scholes, “Dinitrogen Coupling to a Terpyridine-Molybdenum Chromophore Switched on by Fermi-Resonance” Chem 2019, 5, 402-416.
(4) Chanelle C. Jumper, Paul Arpin, Scott McClure, Shahnawaz Rafiq, Jacob C. Dean, Jeffrey Cina, Phillip Kovac, Tihana Mirkovic & Gregory D. Scholes “Broadband Pump-Probe Spectroscopy Quantifies Ultrafast Solvation Dynamics of Proteins and Molecules” J. Phys. Chem. Lett. 2016, 7, 4722–4731.
(5) Madeline H. Elkins, Ryan Pensack, Andrew H. Proppe, Oleksandr Voznyy, Li Na Quan, Shana O. Kelley, Edward H. Sargent, and Gregory D. Scholes, “Biexciton Resonances Reveal Exciton Localization in Stacked Perovskite Quantum Wells” J. Phys. Chem. Lett. 2017, 8, 3895–3901.
(6) Elliot J. Taffet, Yoann Olivier, Frankie Lam, David Beljonne and Gregory D. Scholes “Carbene–Metal–Amide Bond Deformation, Rather Than Ligand Rotation, Drives Delayed Fluorescence” J. Phys. Chem. Lett. 2018, 9, 1620–1626 (2018).
From Synchronization to Strong Light-Matter Coupling. Synchronization abounds in Nature and engineered systems. It coerces complex systems into unified and remarkable functions and is evident from many examples in the natural world—from the beating of a heart to plumes of flashing fireflies. At the molecular scale, synchronization is difficult to achieve owing to stochastic fluctuations in structure and energy. Even more challenging, synchronization on the quantum scale can yield nonclassical properties, but these properties predominate in fragile states that easily decompose through decoherence. Broadly speaking, a theme underpinning recent work is the question of whether there are principles for robust synchronization that traverse the scales from macroscopic to quantum. One platform for producing and enabling study of extraordinary coherent synchronization is “cavity QED”, where an ensemble of molecules sandwiched between two mirrors couples collectively to the vacuum fluctuations of the radiation field.
Chemical reactivity is often boosted by photoexcitation because the energy of an absorbed photon significantly changes the driving force for transforming reactants to products. When quantifying this change in driving force, it has generally been assumed that the entropy change accompanying photoexcitation of molecular systems is small. Models, such as theories for photoinduced electron transfer, therefore equate the free energy changes from reactants to products with energy changes that can be measured directly using spectroscopy (this is clearly described in the celebrated paper of Rehm and Weller). Generally, therefore, our intuitive view is that population dynamics flow spontaneously “downhill”, where the ordering of states is prescribed by the ladder of electronic energy gaps. Recently, we have predicted that this intuitive connection between the spectroscopic ordering of states and the spontaneity of reactivity (dictated by free energy) breaks down dramatically for strongly coupled polariton states. The exceptionally low (von Neumann) entropy of coherent states arising from strong coupling (the LP state) therefore presents an unforeseen resource.
A key prediction of our hypothesis of the entropic resource of the LP state that strong light-matter coupling enables a much lower excitation photon energy (lower by a few wavewnumbers) to drive the same photoinduced reaction as occurs outside the cavity upon photoexcitation at photon energy. The lower excitation energy is supplemented by a substantial –T∆S term to generate the total driving force. We are working to validate this hypothesis by studying the dynamics of photochemical reactions in cavities tuned to the excitation frequency of the reactant species.
Read more:
(1) Courtney A. DelPo, Bryan Kudisch, Kyu Hyung Park, Saeed-Uz-Zaman Khan, Francesca Fassioli, Daniele Fausti, Barry P. Rand, and Gregory D. Scholes. “Polariton Transitions in Femtosecond Transient Absorption Studies of Ultrastrong Light-Molecule Coupling” J.Phys. Chem. Lett. 11, 2667-2674 (2020).
(2) G. D. Scholes, C. A. DelPo, and B. Kudisch. “Entropy Reorders Polariton States” J. Phys. Chem. Lett. 11, 6389−6395 (2020).
(3) Siwei Wang, Gregory D. Scholes, and Liang-Yan Hsu “Coherent-to-incoherent transition of molecular fluorescence controlled by surface plasmon polaritons” J. Phys. Chem. Lett. 11, 5948−5955 (2020).
(4)Gregory D. Scholes. “Polaritons and excitons: Hamiltonian design for enhanced coherence” Proc. R. Soc. A 476, 20200278 (2020).
(5) Siwei Wang, Ming-Wei Lee, Yi-Ting Chuang, Gregory D. Scholes, and Liang-Yan Hsu. “Theory of molecular emission power spectra. I. Macroscopic quantum electrodynamics formalism” J. Chem. Phys. 153, 184102 (2020)
Photosynthetic solar energy conversion. Photosynthesis occurs on an immense scale across the earth. It provides all of Earth’s oxygen and plays a deciding factor in global trends in climate, etc. Energy from sunlight is absorbed by special molecules, like chlorophyll, that are embedded in proteins, comprising the photosynthetic unit. Hundreds of these “chromophores” (light absorbing molecules) are used to harvest sunlight and direct the excitation energy to nature’s solar cells—proteins called reaction centers. Thus, these light-harvesting complexes compensate for the mismatch between solar irradiance and the optimal rate of reaction center operation.
Why study light harvesting? Through bio-inspiration we can learn how to design clever materials for energy capture, we discover new examples of photophysical processes, we more deeply understand light-initiated chemical dynamics. Incredible examples of light to energy conversion systems are found among the diverse photosynthetic organisms, ranging from tropical plants to crustose coralline red algae that dwell on the sea floor, 20 m under water covered with more than 1 m of ice cover. These examples are fascinating case studies, particularly in chemical physics, with experiments and theories revealing the mechanisms involved in the ultrafast energy transfer processes of light harvesting.
Read more:
(1) Gregory D. Scholes, Graham R. Fleming, Alexandra Olaya-Castro and Rienk van Grondelle, “Lessons from nature about solar light harvesting.” Nature Chem., 2011, 3, 763–774.
(2) Margherita Maiuri, Maria B. Oviedo, Jacob C. Dean, Michael Bishop, Zi. S. D. Toa, Bryan M. Wong,Stephen McGill, Gregory D. Scholes “High Magnetic Field Detunes Vibronic Resonances in Photosynthetic Light Harvesting”. J. Phys. Chem. Lett. 2018, 9, 548–554.
(3) Chanelle C. Jumper, Shahnawaz Rafiq, Siwei Wang and Gregory D. Scholes, “From Coherent to Vibronic Light Harvesting in Photosynthesis”. Curr. Opinion in Chemical Biology2018,47, 39–46.
(4) Tihana Mirkovic, Evgeny E. Ostroumov, Jessica M. Anna, Rienk van Grondelle, Govindjee and Gregory D. Scholes “Light Absorption and Energy Transfer in the Antenna Complexes of Photosynthetic Organisms” Chem. Rev. 2017, 117, 249−293.
(5) Elisabetta Collini, Cathy Y. Wong, Krystyna E. Wilk, Paul M. G. Curmi, Paul Brumer, and Gregory D. Scholes, “Coherently wired light-harvesting in photosynthetic marine algae at ambient temperature.” Nature, 2010, 463, 644–648.
Honors
Chair, Princeton Department of Chemistry, 2020-2023
Fellow of the Royal Society (London) 2019
Editor-in-Chief, Journal of Physical Chemistry Letters 2019-
Director of BioLEC, a DOE Energy Frontier Research Center 2018-2022
Co-Director CIFAR Bio-inspired Solar Energy Program 2019-
Masuhara Lectureship Award, Asian Photochemical Conference 2016
Beijing Institute of Technology Adjunct Professor, China 2016–2020
Swiss Chemical Society Lectureship 2016
Senior Fellow, CIFAR Bio-inspired Solar Energy Program 2015–
Fellow, Royal Society of Chemistry (United Kingdom)
NSERC John C. Polanyi Award 2013
Royal Society of Chemistry Bourke Award 2012
D.J. LeRoy Distinguished Professorship, University of Toronto 2011–2014
Baden-Württemberg & Wissenschaftliche Gesellschaft Guest Professor, University of Freiburg 2012
Visiting Professor, Beijing Institute of Technology 2011–2013
The Raymond and Beverly Sackler Prize in Physical Sciences (Tel Aviv University) 2011
Fellow, Royal Society of Canada (Academy of Science) 2009
Royal Society of Canada Rutherford Memorial Medal in Chemistry, 2007
NSERC Steacie Memorial Fellowship Award, 2007–2009
Chemical Institute of Canada Keith Laidler award, 2006
Alfred P. Sloan Foundation Fellow, 2004–2006
Research Innovation Award (Research Corporation) 2002
Premier of Ontario’s Research Excellence Award 2000
Ramsay Memorial Postdoctoral Research Fellow, 1995–1997
Selected Publications
Gregory D. Scholes; Entropy, 25, 1519 (2023).“ Large Coherent States Formed from Disordered k-Regular Random Graphs”
Aarat Kalra; Alfy Benny; Sophie M. Travis; Eric A. Zizzi; Austin Morales-Sanchez; Daniel D. Oblinsky; Travis J. A. Craddock; Stuart R. Hameroff; M. Bruce MacIver; Jack A. Tuszynski; Sabine Petry; Roger Penrose; Gregory D. Scholes, ACS Central Science, 9, 352-361 (2023). “Electronic Energy Migration in Microtubules”
Xinzi Zhang; Kyra N. Schwartz; Luhao Zhang; Francesca Fassioli, Bo Fu; Lucas O. Nguyen; Robert R. Knowles; Gregory Scholes, Proc. Natl. Acad Sci USA, 119, 43 (2022).“ Interference of nuclear wavepackets in a pair of proton transfer reactions”
Xinizi Zhang; Keyu Geng; Donglin L. Jiang; Gregory D. Scholes, Journal of the American Chemical Society, 144, 36, 16423-16432, (2022). “Exciton Diffusion and Annihilation in an sp(2) Carbon-Conjugated Covalent Organic Framework”
D. Earley; A. Zieleniewska; H.H. Ripberger; N.Y. Shin; M.S. Lazorski; Z. J. Mast; H. J. Sayre; J. K. McCusker; Gregory D. Scholes; Robert R. Knowles; O.G. Reid; G. Rumbles, Nature Chemistry, 14, 7, 746+ (2022). “Ion-pair reorganization regulates reactivity in photoredox catalysts”
Gregory D. Scholes, Proceeding of the Royal Society A; 478, 2265 (2022). “The Kuramoto-Lohe model and collective absorption of a photon”
Courtney A. DelPo; Sarah E. Bard; Gregory D. Scholes, Journal Of Physical Chemistry C (2022). “Annealing Controls Ultrafast Dynamics of Carrier Production in Organic Photovoltaics Incorporating a Nonfullerene Acceptor”
JunWoo J. Kim; Tu C. Nguyen-Phan,; Gardiner, Alastair T.; Tai Hyun Yoon; Richard J. Cogdell; Minhaeng; Gregory D. Scholes., Journal of Physical Chemistry Letters 13, 4, 1099-1106 (2022). “Vibrational Modes Promoting Exciton Relaxation in the B850 Band of LH2”
Somnath Biswas; JunWoo Kim; Xinzi X. Zhang; Gregory D. Scholes; Chemical Reviews; 122, 3, 4257-4321 (2022). “Coherent Two-Dimensional and Broadband Electronic Spectroscopies”
Hannah Sayre, Lei Tian, Minjung Son, Steph Hart, Xiao Liu, Daniela Arias-Rotondo, Barry Rand, Gabriela Schlau-Cohen, Greg Scholes. “Solar Fuels and Feedstocks: The Quest for Renewable Black Gold” Energy & Environmental Science 14, 1402-1419 (2021).
Elliot J. Taffet, David Beljonne, and Gregory D. Scholes. “Overlap-Driven Splitting of Triplet Pairs in Singlet Fission” Journal of the American Chemical Society 2020, 142, 47, 20040-20047
Robert Kirby, Austin Ferrenti, Caroline Weinberg, Sebastian Klemenz, Mohamed Oudah, Chris Weber, Daniele Fausti, Gregory D. Scholes, and Leslie Schoop. “Transient Drude Response Dominates Near-Infrared Pump-Probe Reflectivity in Nodal-Line Semimetals ZrSiS and ZrSiSe”. J. Phys. Chem. Lett. 11(15), 6105-6111 (2020).
Nicholas Till, Lei Tian, Zhe Dong, Gregory D. Scholes, and David WC MacMillan. “A Mechanistic Analysis of Metallaphotoredox C–N Coupling: Photocatalysis Initiates and Perpetuates Ni(I)/Ni(III) Coupling Activity” J. Am. Chem. Soc. 142(37), 15830-15841 (2020).
Bryan Kudisch, Margherita Maiuri, Luca Moretti, Maria B. Oviedo, Leon Wang, Daniel G. Oblinsky, Robert K. Prud’homme, Bryan M. Wong, Stephen A. McGill, and Gregory D. Scholes. “ Ring currents modulate optoelectronic properties of aromatic chromophores at 25 T” Proc. Natl. Acad. Sci. U.S.A. 117, 11289-11298 (2020).
Stephen Ting, Sofia Garakyaraghi, Chelsea Taliaferro, Benjamin Shields, Gregory D. Scholes, Felix Castellano, and Abigail Doyle “3D-D Excited States of Ni(II) Complexes Relevant to Photoredox Catalysis: Spectroscopic Identification and Mechanistic Implications” J. Am. Chem. Soc. 142(12), 5800-5810 (2020).
Kyle Biegasiewicz, Simon J. Cooper, Xin Gao, J Kim, Daniel G. Oblinsky, Braddock Sandoval, Gregory D. Scholes, Todd K. Hyster, “Photoexcitation of a Flavoenzyme Enables a Stereocontrolled Radical Cyclization” Science. 364, 1166–1169 (2019).
Margherita Maiuri, Maria B. Oviedo, Jacob C. Dean, Michael Bishop, Zi. S. D. Toa, Bryan M. Wong, Stephen McGill, Gregory D. Scholes “High Magnetic Field Detunes Vibronic Resonances in Photosynthetic Light Harvesting” J. Phys. Chem. Lett.. 9, 548–554 (2018).
Chanelle C. Jumper, Shahnawaz Rafiq, Siwei Wang and Gregory D. Scholes, “From Coherent to Vibronic Light Harvesting in Photosynthesis” Curr. Opinion in Chemical Biology 47, 39–46 (2018).
Ryan D. Pensack, Andrew J. Tilley, Christopher Grieco, Evgeny E. Ostroumov, Geoffrey E. Purdum, Devin B. Granger, Daniel G. Oblinsky, Jacob C. Dean, John B. Asbury, Yueh-Lin Loo, Dwight S. Seferos, John E. Anthony, and Gregory D. Scholes, “Striking the right balance of intermolecular coupling for high-efficiency singlet fission” Chem. Sci. 9, 6240-6259 (2018).
Margherita Maiuri, Evgeny E. Ostroumov, Rafael G. Saer, Robert E. Blankenship, Gregory D. Scholes, “Coherent Wavepackets in the FMO Complex are Robust to Spectral Perturbations by Mutagenesis” Nature Chem. 10, 177–183 (2018).
Jacob, C. Dean & Gregory D. Scholes, “Coherence Spectroscopy in the Condensed Phase: Insights into Molecular Structure, Environment, and Interactions” Acc. Chem. Res. 50, 2746–2755 (2017).
Jacob C. Dean, Tihana Mirkovic, Zi S. D. Toa, Daniel Oblinsky & Gregory D. Scholes “Vibronic Enhancement of Algae Light Harvesting” Chem (Cell Press) 1, 858–872 (2016).
Elsa Cassette, Ryan D. Pensack, Benoît Mahler and Gregory D. Scholes, “Room-Temperature Exciton Coherence and Dephasing in Two-dimensional Nanostructures” Nature Comm. 6, 6086 (2015).
Evgeny E. Ostroumov, Rachel M. Mulvaney, Richard J. Cogdell, Gregory D. Scholes “Broadband 2D Electronic Spectroscopy Reveals a Carotenoid Dark State in Purple Bacteria” Science 340, 52–56 (2013).
Elisabetta Collini, Cathy Y. Wong, Krystyna E. Wilk, Paul M. G. Curmi, Paul Brumer, and Gregory D. Scholes, “Coherently wired light-harvesting in photosynthetic marine algae at ambient temperature” Nature 463, 644–648 (2010).
Jeongho Kim, Vanessa M. Huxter, Carles Curutchet, Gregory D. Scholes, “Measurement of Electron-Electron Interactions and Correlations using Two-Dimensional Electronic Double-Quantum Coherence Spectroscopy” J. Phys. Chem. A 113, 12122–12133 (2009).
Marcus Jones, Shun S. Lo, & Gregory D. Scholes, “Quantitative modeling of the role of surface traps in CdSe/CdS/ZnS nanocrystal photoluminescence decay dynamics” Proc. Natl. Acad. Sci. USA 106, 3011–3016 (2009).
Vanessa M. Huxter, Anna Lee, Shun S. Lo, & Gregory D. Scholes, “CdSe nanoparticle elasticity and surface energy” Nano Letters 9, 405–409 (2009).
Cathy Y. Wong, Jeongho Kim, P. Sreekumari Nair, Michelle C. Nagy & Gregory D. Scholes, “Relaxation in the exciton fine structure of semiconductor nanocrystals” J. Phys. Chem. C 113, 795–811 (2009). Feature Article.
Gregory D. Scholes, Jeongho Kim, & Cathy Y. Wong, “Exciton spin relaxation in quantum dots measured using ultrafast transient polarization grating spectroscopy” Phys. Rev. B 73, 195325 (13 pages) (2006).
Related News
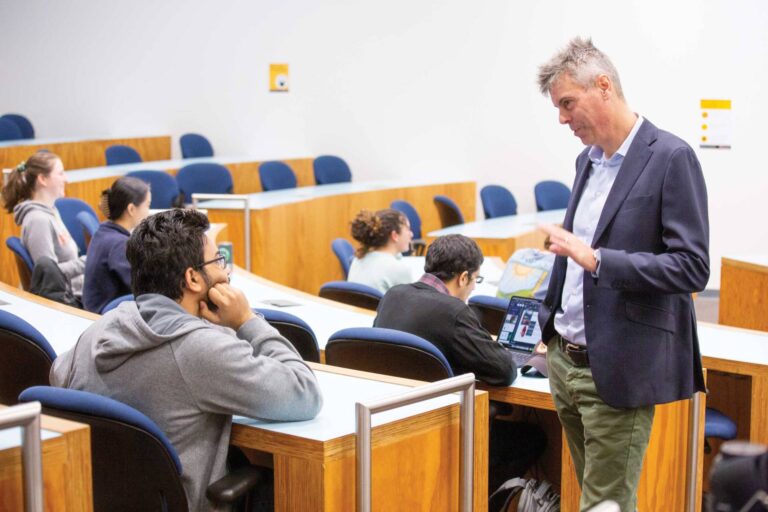
Scholes/Weichman Teach New Approach to the Quantum World in CHM 305
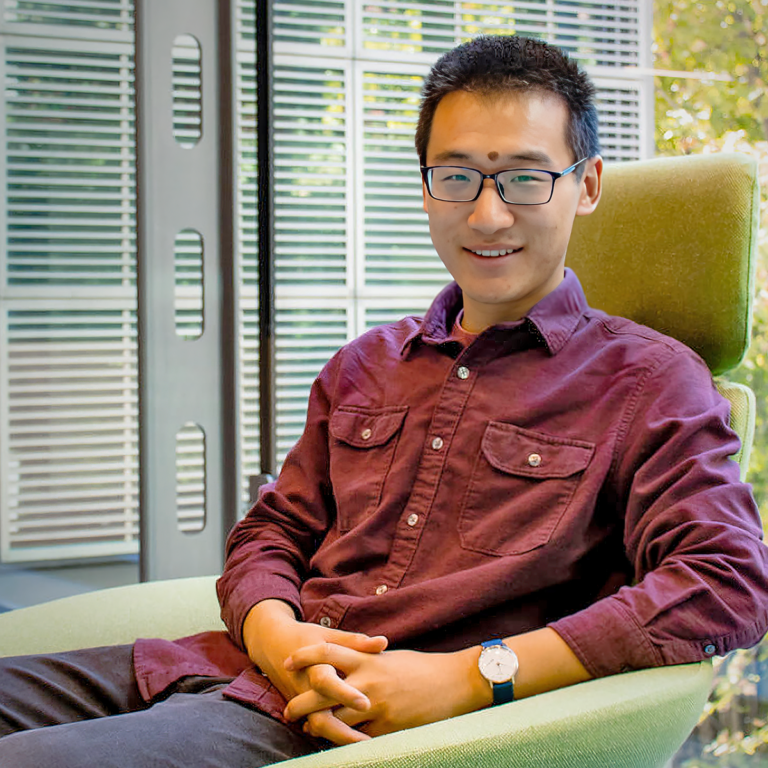