
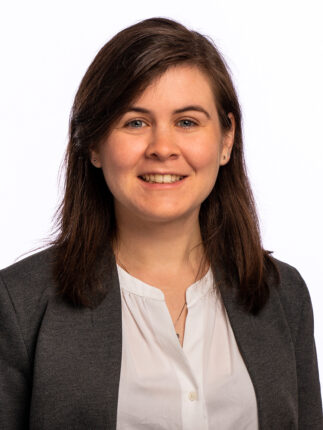
Marissa Weichman
Contact:
Weichman Lab Website
Marissa L. Weichman
Assistant Professor of Chemistry
[email protected]
Frick Laboratory, 229
609-258-0926
Faculty Assistant:
Julia Wlodarczyk-Mingozzi
Faculty / Grants Assistant
[email protected]
Frick Laboratory, 389
609-258-3674
Research Focus
The Weichman Lab uses fundamental chemical physics and spectroscopy to probe the detailed behavior of complex chemical systems and develop new ways to steer molecular processes using light.
Controlling Molecular Processes with Strong Light-Matter Interactions
Achieving rational photonic control of chemical reactions is a long-standing goal of physical chemistry. The emerging field of polariton chemistry may soon provide a new architecture to achieve this goal by harnessing strong light-matter interactions engineered in optical cavities. Polaritons are mixed light-matter states that arise when the confined electromagnetic field of an optical cavity interacts strongly with a bright transition of a molecular ensemble. A considerable body of experimental and theoretical work has already demonstrated that strong coupling can dramatically alter intracavity molecular processes. However, the mechanisms underlying cavity-altered chemistry remain unclear in large part because the experimental systems examined previously are too complex for detailed analysis of their reaction dynamics. Deriving the governing set of rules for chemistry under strong coupling is a necessary first step before we can construct practical polaritonic reactors.
Our lab is establishing a new body of experimental work to validate theories of cavity-modified chemistry. These problems demand surveys of computationally-tractable, strongly-coupled reactions with experimental methods that can directly track the time-dependent populations of reactants, transient intermediates, and products in specific quantum states. We use both ultrafast and high-resolution spectroscopy to follow the dynamical trajectories of benchmark solution-phase and gas-phase molecular processes under strong coupling. Our measurements inform prospects for using the new degrees of freedom afforded by cavity coupling to direct increasingly complex chemistry
Precision Spectroscopy of Extremely Large Molecules
Precision spectroscopy is an essential tool to assign molecular fingerprints in complex environments including Earth’s atmosphere and interstellar space; to inform molecular control schemes for applications in quantum information science; and to benchmark state-of-the-art quantum chemistry methods. While detailed spectroscopy is routine for small molecules, resolving individual quantum states in systems with 50 or more atoms lies on the brink of treatment with our current toolkit. Quantum-state-resolved spectroscopy of large molecules demands a method with excellent frequency resolution, extremely high sensitivity, broadband readout, and rapid acquisition times. Optical frequency comb lasers are the only existing technology that can simultaneously meet these demands. The spectra of these light sources consist of thousands of narrow, evenly spaced “comb teeth,” essentially acting as many thousands of individual stable lasers lasing synchronously. In cavity-enhanced frequency comb spectroscopy, we match a comb’s spectral lines to the resonant modes of an optical cavity containing the absorber of interest. The cavity dramatically increases the pathlength of light through the sample, yielding highly sensitive absorption spectra. Our lab is developing a first-of-its-kind rapid-acquisition cavity-enhanced frequency comb spectrometer working in the long-wave infrared.
Our research interests for comb spectroscopy lie in large part in laboratory astrochemistry: the detection of new astrochemical species is key to understanding the chemical underpinnings of star, planet, and galaxy formation and the origins of prebiotic molecules. We are targeting quantum-state-resolved spectroscopic studies of fullerenes and polycyclic aromatic hydrocarbons to aid in the identification of these large, complex species in observational data. Along the way, our work will establish new records in the complexity and size of molecular systems that can be examined with complete quantum state resolution.
New Spectroscopic Tools for Single-Particle Aerosol Science
Atmospheric aerosols have an outsized influence on Earth’s climate through their interactions with light and clouds. These nanometer-scale particles scatter and absorb incoming sunlight as well as outgoing terrestrial radiation. The relative contributions of these optical processes underpin aerosols’ direct impacts on the radiative balance of our atmosphere – the ratio of solar energy absorbed to energy emitted that, when tipped, drives the climate out of equilibrium. Aerosols also nucleate cloud droplets, influencing local droplet densities, size distributions, and lifetimes which cause cascading indirect effects on climate and weather. Aerosol-light and aerosol-cloud interactions remain the largest sources of uncertainty in our best models of Earth’s radiative balance. Our current knowledge of these intricate systems is not sufficient to quantitatively capture their role in the climate crisis.
To make progress, we must clarify precisely how aerosols of different size and composition scatter and absorb light and nucleate cloud droplets. We must additionally determine how aging, wetting, and chemical processing of particles changes their behavior over time. Our lab is advancing the frontier of state-of-the-art laboratory spectroscopies to interrogate this detailed atmospheric aerosol microphysics. To correlate aerosol size and composition with optical behavior, we perform spectroscopy on a well-defined sample: the isolated single particle. We are harnessing broadband cavity-enhanced spectroscopy for measurements of the optical properties, droplet nucleation dynamics, and chemical aging of electrodynamically-trapped atmospherically-relevant aerosols.
Honors
Cottrell Scholar Award 2025
Presidential Early Career Award for Scientists and Engineers 2025
Packard Fellowship for Science and Engineering 2023
NSF CAREER 2023
DOE Early Career Award 2022
ACS PRF Doctoral New Investigator 2021
NIST/NRC Postdoctoral Research Fellow 2017-2019
APS Justin Jankunas Doctoral Dissertation Award in Chemical Physics 2018
NSF Graduate Research Fellow 2012-2017
Selected Publications
A. P. Fidler, L. Chen, A. M. McKillop, M. L. Weichman. Ultrafast dynamics of CN radical reactions with chloroform solvent under vibrational strong coupling. J. Chem. Phys. 159, 164302 (2023).
A. D. Wright, J. C. Nelson, M. L. Weichman. A versatile platform for gas-phase molecular polaritonics. J. Chem. Phys. 159, 164202 (2023).
A. D. Wright, J. C. Nelson, M. L. Weichman. Rovibrational polaritons in gas-phase methane. J. Am. Chem. Soc. 145, 5982-5987 (2023).
J. H. Lehman and M. L. Weichman. Optical frequency combs for molecular spectroscopy, kinetics, and sensing. Emerging Trends in Chemical Applications of Lasers, Ch. 4, pp. 61-88 (2021).
P. B. Changala, M. L. Weichman, K. F. Lee, M. E. Fermann, and J. Ye. Rovibrational quantum state resolution of the C60 fullerene. Science 363, 49-54 (2019).
M. L. Weichman, P. B. Changala, M. Yan, N. Picqué, and J. Ye. Broadband molecular spectroscopy with optical frequency combs. J. Mol. Spec. 355, 66-78 (2019).
M. L. Weichman and D. M. Neumark. Slow photoelectron velocity-map imaging spectroscopy of cryogenically cooled anions. Annu. Rev. Phys. Chem. 69, 101-124 (2018).
J. A. DeVine, M. L. Weichman, B. Laws, J. Chang, M. C. Babin, G. Balerdi, C. Xie, C. L. Malbon, W. C. Lineberger, D. R. Yarkony, R. W. Field, S. T. Gibson, J. Ma, H. Guo, and D. M. Neumark. Encoding of vinylidene isomerization in its anion photoelectron spectrum. Science 358, 336-339 (2017).
M. L. Weichman, J. A. DeVine, M. C. Babin, J. Li, J. Ma, H. Guo, and D. M. Neumark. Feshbach resonances in the exit channel of the F + CH3OH → HF + CH3O reaction observed using transition-state spectroscopy. Nat. Chem. 9, 950-955 (2017).
M. L. Weichman, J. A. DeVine, D. S. Levine, J. B. Kim, and D. M. Neumark. Isomer-specific vibronic structure of the 9-, 1-, and 2-anthracenyl radicals via slow photoelectron velocity-map imaging. Proc. Natl. Acad. Sci. U.S.A. 113, 1698-1705 (2016).
J. B. Kim, M. L. Weichman, T. F. Sjolander, D. M. Neumark, J. Kłos, M. H. Alexander, and D. E. Manolopoulos. Spectroscopic observation of resonances in the F + H2 reaction. Science 349, 510-513 (2015).
M. L. Weichman, J. B. Kim, J. A. DeVine, D. S. Levine, and D. M. Neumark. Vibrational and electronic structure of the α- and β-naphthyl radicals via slow photoelectron velocity-map imaging. J. Am. Chem. Soc. 137, 1420-1423 (2015).
Related News

Schoop, Weichman Receive Biden Administration PECASE Awards
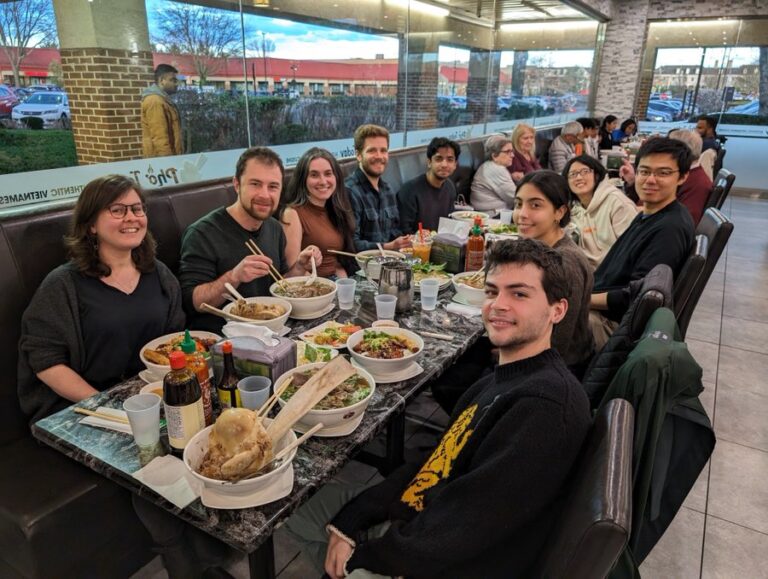
Building a Lab out of Thin Air: Weichman, Roque, and Kunin
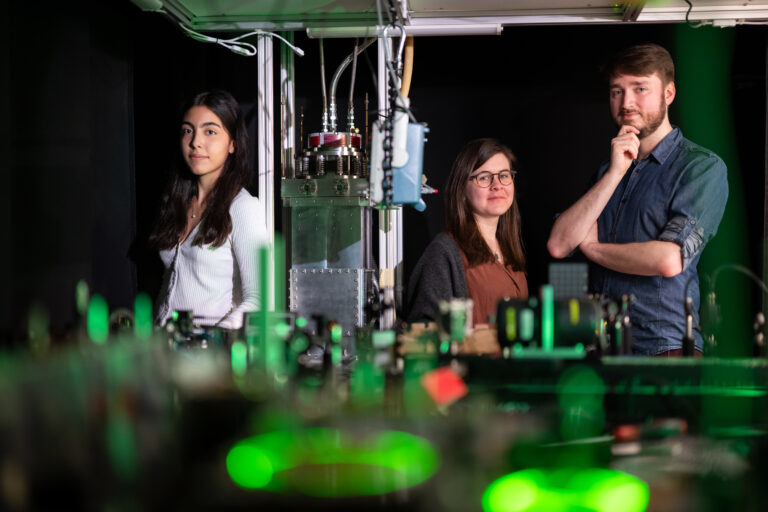