Hyster and MacMillan Report New Platform for Stereocontrol
A collaboration between two Department of Chemistry labs has yielded a striking new platform that allows chemists to reinterpret the rules of stereochemistry and stereocontrol with important implications for the pharmaceutical and agrochemical industries.
The research demonstrates the ability of photoredox catalysis to take traditionally static stereocenters and render them dynamic by continuously and controllably breaking and re-forming molecular bonds. It shows promise for scientists to set the stereocenters of a complex target compound in a succinct, efficient step rather than one stereocenter at a time. (A stereocenter gives a molecule mirror-image asymmetry, which is important in determining biologically active properties during synthesis.)
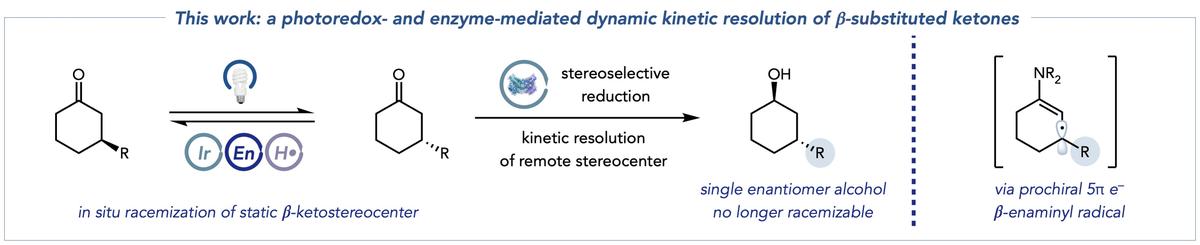
Illustration by Jacob DeHovitz
The collaboration pairs expertise from the MacMillan lab and the Hyster lab. The paper, “Static to Inducibly Dynamic Stereocontrol: The Convergent Use of Racemic β-Substituted Ketones,” appears in Science this week.
“Normally, when you’re thinking about building molecules with stereocenters in them, you think about setting the stereocenter in the bond-forming event,” said Todd Hyster, assistant professor of chemistry. “And what we’ve found is that if you use photoredox catalysis, you can essentially unlock mechanisms to make stereocenters that would otherwise be static, or fixed. You can render them dynamic.
“When you couple those with highly selective enzymes, you can think about building stereochemically complex molecules in completely new ways.”
Stereochemistry involves the spatial arrangement of atoms and how they can be manipulated in the molecular structure for desired outcomes. It derives from the fact that carbon has four substituents. Those four substituents are different; they have to exist as mirror images of each other. These mirror images are called enantiomers. Our hands are enantiomers, for example; they are mirror images of each other. What that means practically is that they cannot be superimposed – a left-hand glove won’t fit on a right hand.
The human body responds to medicines in exactly the same way. Our biological matter is made up of one series of mirror images and not the other. The body can recognize the difference between two medicines that appear identical even though one is a mirror image and one is not. That recognition influences the uptake and therefore the efficacy of therapies.
What the MacMillan and Hyster research uncovered is a way to decouple the configuration of the stereocenter from the step in which it is formed, or racemized. Being able to control this racemization is important to the development of the most effective medicines.
“There are many, many reasons why this is exciting research, not least of which is you can basically make a molecule and you can say, maybe I’d really like to change that stereocenter to make it into something else. You can actually go back and do that,” said David MacMillan, the James S. McDonnell Distinguished University Professor of Chemistry. “This is something which we don’t think has really been done this way before in organic chemistry and so it opens up some remarkable opportunities.”
Enzymes Boost the Results
It was the introduction of highly selective enzymes into the process that drove the investigation’s advances from “B+ to A+” research, MacMillan said.
“Enzymes have this way of being able to impart what’s called stereocontrol, or introducing very specific shapes to do chemistry that ordinary catalysts just can’t do,” he said. “By bringing these two ideas together, it allows a completely new approach to how you build stereochemistry.”
There’s a lot going on under the hood in this process. When scientists synthesize a compound with several stereocenters, they make the assumption that the center cannot flip or become dynamic once it is “set.” Molecules are synthesized sequentially: a center is formed, and then it’s locked; and then another is formed, and then it’s locked, and so on.
Jacob DeHovitz, a fifth-year graduate student in the Hyster lab and lead author on the paper, explained how the reaction described in this research enables scientists to unlock that setting.
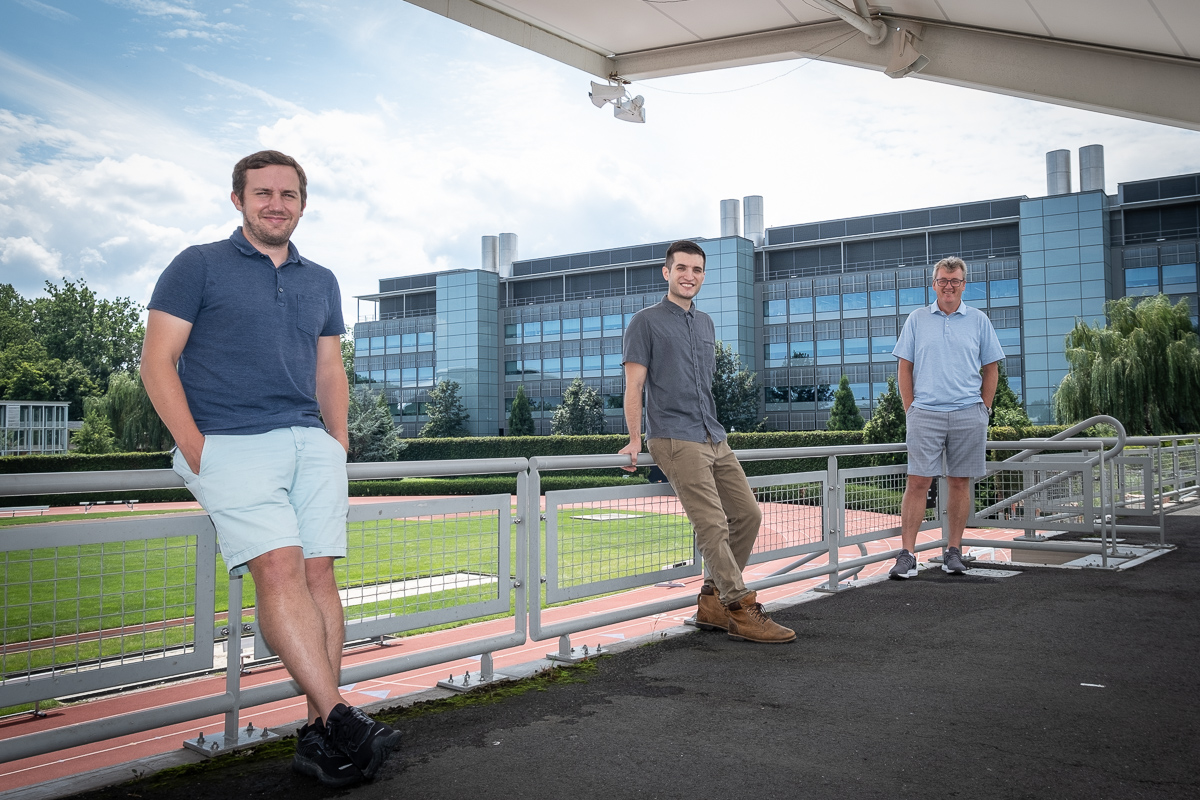
Photo by C. Todd Reichart
There are two important parts to the reaction. In the first half, two key species are formed from a ketone starting material. The first species is called an enamine, key because it can be targeted by the photoredox catalyst. A second species – an enaminyl radical – results from that step, and destroys the original molecular stereocenter and sets up the next step in the process.
“The second half is the biocatalytic reaction. The enzyme in solution – in this case, mainly ketoreductases – reduces one of those ketone enantiomers, which again are constantly racemizing in solution, to form an alcohol product that cannot undergo racemization under our conditions. Critically, the other ketone enantiomer does not react with the ketoreductase because it cannot fit into the active site of the enzyme. This bias for one enantiomer is important because it prevents the ketoreductase from the undesirable formation of other alcohol stereoisomers.
“If the biocatalytic reaction is combined with the novel racemization conditions where the starting material enantiomers are constantly racemizing, then a chemist can theoretically afford up to 100% of the desired product, doubling the efficiency,” DeHovitz said.
This research represents three years of collaborative investigation by the two labs. Read the full paper here: https://science.sciencemag.org/content/369/6507/1113
“Static to Inducibly Dynamic Stereocontrol: The Convergent Use of Racemic β-Substituted Ketones,” DOI 10.1126/science.abc9909. Research reported in this paper was supported by BioLEC, an Energy Frontier Research Center funded by the U.S. Department of Energy, Office of Science under award #DESC0019370, and the Princeton Catalysis Initiative. Authors are affiliated with the Merck Center for Catalysis: J.S.DH., Y.Y.L., J.A.K., K.N., A.J.M., M.Y., D.W.C.M., T.K.H.