Jacobs Intros Model to Quantify Nonequilibrium Assembly Kinetics
The kinetics behind molecular self-assembly at equilibrium is governed by a well-established set of physical rules. What is less clear to scientists is how the rules change as a system moves away from equilibrium, and whether the kinetics are similar in living systems.
As part of its mission to demystify this process, the Jacobs Group introduces a theoretical model to quantify nonequilibrium assembly kinetics, contributing key insights by driving a system out of equilibrium and then measuring the results.
Using their model, researchers were able to either accelerate or suppress the rate of nucleation under different conditions by altering the interfacial surface tension. Nucleation occurs within a living system when biomolecules gather, or assemble, to form a condensate that can then carry out a biological function.
The group’s paper, Tuning Nucleation Kinetics via Nonequilibrium Chemical Reactions, was published last week in Physical Review Letters.
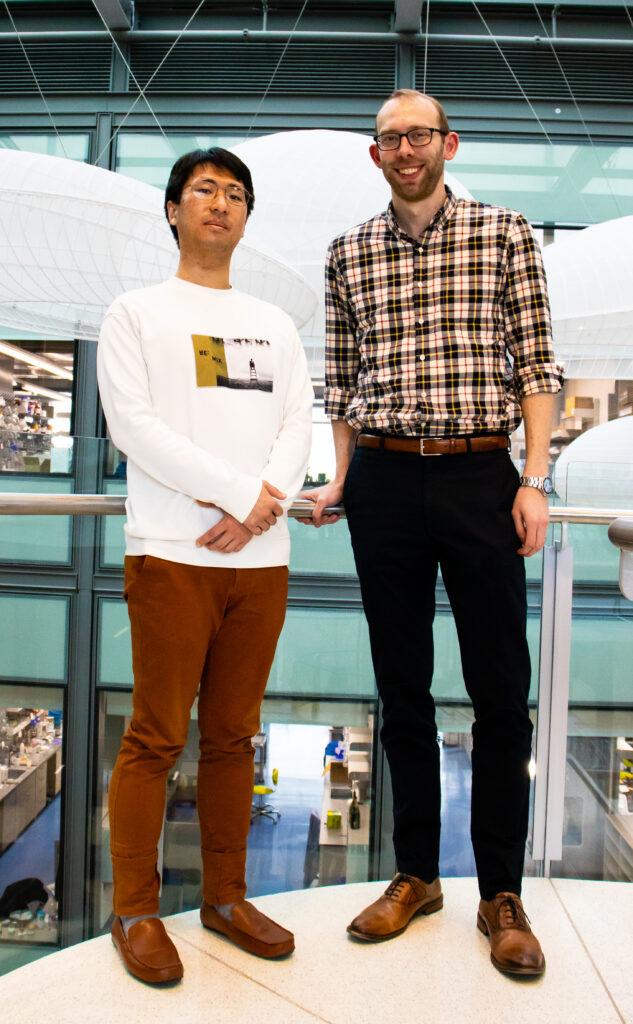
Authors on the new Jacobs Lab research, l to r: Yongick Cho, a fourth-year graduate student; and Assistant Professor William Jacobs.
“The general understanding in the field is that biomolecular condensate assembly, due to the physical principle of phase separation, is very much like oil and water demixing. Oil droplets will form. That’s a thermodynamically driven process,” said William Jacobs, assistant professor of chemistry. “But of course, living cells are not simple materials in the same way that oil and water are, so there are many open questions here. How could phase separation potentially be different in liquid-like phases of living materials? And if it is different, how would you know? What would you measure?
“What we’ve shown here is a particular mechanism for how the kinetics of this phase transformation can change and what ingredients are required to change them relative to what you have in equilibrium. The takeaway message is really about the mechanism – identifying what you need to change the nucleation rate by driving the system out of equilibrium.”
Thermodynamic equilibrium means there is no net transfer of energy into a system. But living cells need energy to carry out their functions, so they draw energy from outside. This energy exchange is the key factor that drags a system out of equilibrium.
What the Jacobs Group found is that driving a system out of equilibrium can change the surface tension associated with a phase transition and thus alter the nucleation kinetics.
As part of the research, Yongick Cho, a fourth-year graduate student in the group, developed a theory that can predict whether the surface tension will increase or decrease depending on certain chemical reaction-rate choices, providing “experimental knobs” that can be turned to circumvent the rules of equilibrium thermodynamics.
He used a lattice gas model—a well-studied model for explaining how phase separation happens in equilibrium fluids—and tweaked it to allow the molecular species that want to phase separate to exist in either one of two conformational states.
Then, by adding a chemical reaction, Cho was able to shift the population of molecules into one state or the other.
“We know that the rate of nucleation can happen much faster than is expected in equilibrium, or the absence of energy from the outside,” said Cho. “If you divide the system into the inside of the nucleated system and anything outside of it, then there is a surface that separates the two regions of the cell,” said Cho. “The surface tension between them is the energy associated with that interface. If the value of the surface tension is large, it is hard to grow self-assembled structures.
“So in our model, we show that nonequilibrium chemical reactions can lower that surface tension value so that the kinetics become much faster. If you know the relation and how it’s specified—meaning, the energy input from the outside—then we can automatically know how fast the self-assembly happens. And that allows us to tune the process.”
The research builds on the work being done elsewhere at Princeton University on biomolecular condensates and the physical principles underlying their organization, as with for example the Clifford Brangwynne Lab in the Department of Chemical and Biological Engineering.
Jacobs said he was “thrilled” with Cho’s results, obtained after three years of work.
“I think the community will find this very interesting,” said Jacobs. “Hopefully, it will inspire other people to look for different types of material and interfacial properties that are affected by dragging a system out of equilibrium.”
Read the Jacobs Group paper in Physical Review Letters.
This research was supported by the National Science Foundation (DMR-2143670).