New method of molecular coupling to expedite drug discovery process
Bringing new drugs to market takes time. The process, which includes laboratory testing, clinical research, and FDA review, is lengthy. According to most estimates documented in the literature, it takes an average of 17 years for research evidence to reach clinical practice.
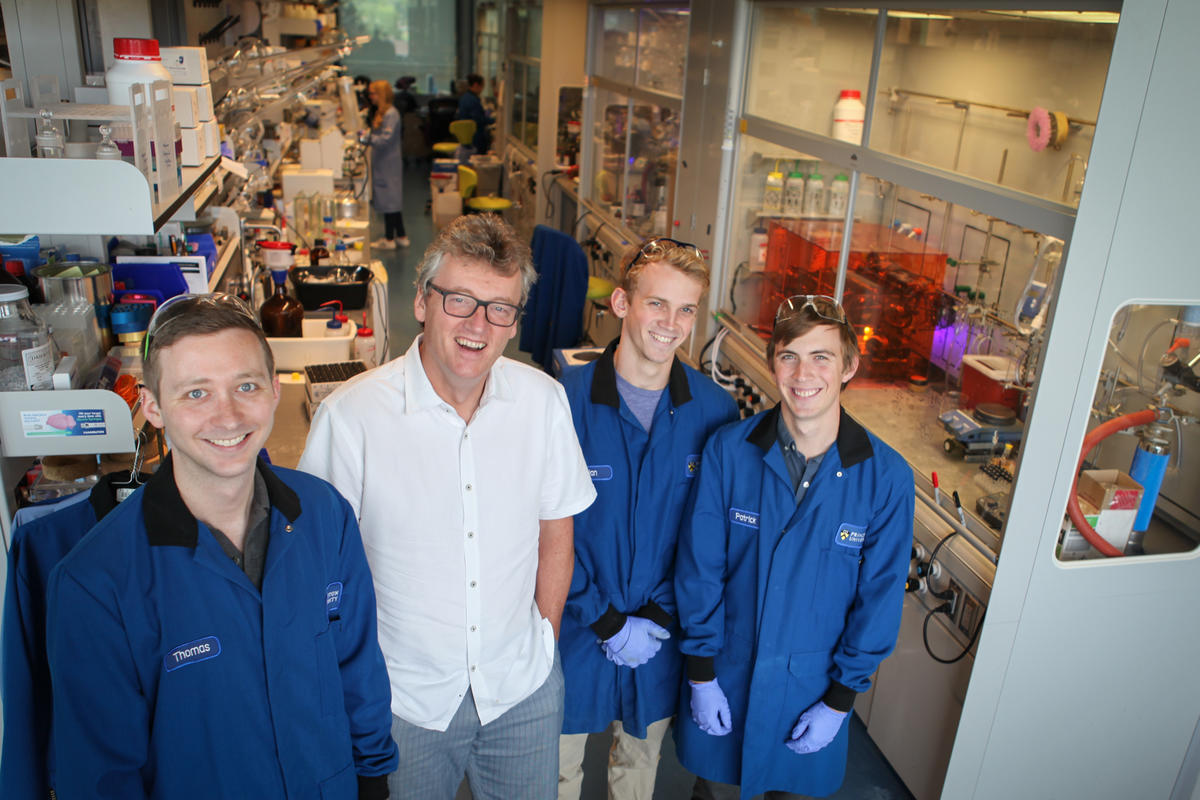
But working alongside Merck, researchers in David MacMillan’s lab at Princeton have found new ways to bond molecules—coupling aliphatic carbon frameworks with a range of aryl bromide coupling partners—using a combination of light-driven, polyoxometalate-facilitated hydrogen atom transfer (HAT) and nickel catalysis. This illustrates an important and previously elusive synthetic transformation, paving the way for the development of related chemical reactions. Further, the real-world application of this technology could mean an expedited drug development process, and down the line, make pharmaceutical therapies available to clinicians and their patients in a shortened time frame.
The early-stage work done by medicinal chemists is fundamental to the drug discovery process. Laboratory research on refining molecules, changing their structure and shape so they behave in predictable ways, is labor intensive and often has a long timeline—up to 7-8 years.
At the end of that window, if lead molecules work on a biological target, the outcome is a medicine. Still then, it must move through the regulatory process—adding even more time until a drug is brought to market.
Further, this behind-the-scenes work of medicinal chemists can involve tedious testing of thousands of different molecules in order to find a suitable candidate for clinical investigation.
But what if organic chemistry researchers could speed up that process, by providing medicinal chemists with new tools that would facilitate selective molecule activation to support drug discovery? “We invent completely new chemical reactions that are hopefully going to have a large impact on medicinal chemists,” says David MacMillan, James S. McDonnell Distinguished University Professor of Chemistry and Director of the Merck Center for Catalysis at Princeton.
Not only does their work provide a new tool to support drug discovery but it promises time-saving efficiency, which can translate into economic and therapeutic value for the pharmaceutical industry and the patients it serves.
The work of MacMillan’s research group, documented in its paper in this week’s edition of Nature, offers new ways to “tremendously streamline ways to bond molecules, but also make new molecular couplings—it’s like LEGO,” he explains, using the popular building toy as an accessible analogy.
Whereas prior work in this area provided powerful methods to directly functionalize specific kinds of electronically-distinct C–H bonds (generally electron rich or “hydridic” C–H bonds adjacent to either oxygen or nitrogen atoms), Patrick Sarver, graduate researcher and one of the paper’s co-authors describes the novel aspects of the group’s targeted method.
“[It] provides a direct route to react with C–H bonds that truly lack any adjacent ‘activating’ functionality. This enables direct derivatization of molecules at positions that would be completely unreactive under previously published conditions, further expanding the range of interesting, complex molecules organic chemists can make from simple starting materials in a single step,” says Sarver.
“We believe this method will be extremely useful to chemists who seek to develop efficient routes to a broad range of highly three-dimensional, arylated products from simple starting materials. In our paper, the one-step synthesis of protected epibatidine and a range of analogues, all part of a previously investigated class of promising non-opioid pain relievers, illustrates the direct application of this new technology to the rapid synthesis of a range of medicinally-relevant molecules,” he says.
“Additionally, the C(sp3)–C(sp2) bonds formed in this reaction are particularly challenging to make using more traditional cross-coupling methodologies. The presence of such bonds has recently proven to be of great interest in drug discovery, as increased C(sp3) content correlates positively with clinical success.”
Sarver notes how this should enable medicinal chemists to explore molecules that they might otherwise be unable to make, some of which could eventually enter clinical trials and ultimately become drugs.
“By combining the selective hydrogen atom abstraction of decatungstate with the generality of nickel catalyzed cross-coupling reactions, our reaction will enable medicinal chemists to selectively introduce a range of aromatic fragments at specific locations on complex, three-dimensional scaffolds, beginning from simple, unfunctionalized starting materials.”
“Doing so will enable the rapid determination of structure-activity relationships in a part of chemical space that is currently under-explored due to synthetic difficulties forming this type of carbon-carbon bond,” he says.
Since the group’s reaction only requires a simple C–H bond as one of the coupling partners, it can improve synthetic efficiency by reducing the need to “prefunctionalize” the starting material. This translates into a reduction of preliminary steps which in turn increases the speed at which medicinal chemists can deliver new molecules for testing.
“First, from a synthetic standpoint, our new reaction provides access to a range of complex, medicinally relevant products that are currently extremely challenging to access. This has the power to enable medicinal chemists to generate a wide range of closely related compounds on scaffolds that they might otherwise be unable to investigate and could thus help to speed up the long and difficult process of drug discovery,” he says.
Throughout the last several decades, the development of new, milder partners for cross-coupling reactions has been a major field of research—with work on palladium-catalyzed cross couplings by Heck, Negishi, and Suzuki receiving the 2010 Nobel Prize for Chemistry.
But this work from MacMillan’s group represents the first time completely unactivated C–H bonds have been found to function as effective partners in a general cross-coupling reaction. “By directly reacting with an unactivated C–H bond, we remove the need for those additional “prefunctionalization” steps, thereby allowing chemists to take an extremely simple starting material and immediately and selectively introduce complexity. The C–H bonds functionalized in our reactions are, fundamentally, the ideal coupling partners: they are both widely abundant and unreactive under almost all reaction conditions,” Sarver says.
In summary, this work from MacMillan’s group offers a way to leverage the modularity of more traditional cross-coupling methodologies and combine it with the efficiency and generality of hydrogen atom transfer-mediated C–H functionalization.
And as MacMillan himself notes, one of the many valuable things about this work is anchored in its genesis as an academic-industrial collaboration. While the project was developed from an academic approach, with its own disciplinary standards of intellectual rigor, it had a clearly-defined end user in mind. Conducting front line research with defined target outcomes was valuable and offered a symbiotic connection, said MacMillan.
While the group’s work doesn’t make a drug, it does provide a tool that offers a faster way to get to the finish line. MacMillan described their contribution using this analogy: this method of constructing new molecular couplings and reactions is like replacing a lone carpenter with a whole construction crew. Not only can the house—that is, the drug—be built faster that way, but for medical chemists, using this technology extends the possibility of building new and different types of “houses” in the future.
The paper, Direct Arylation of Strong Aliphatic C-H Bonds, by Ian B. Perry, Thomas F. Brewer, Patrick J. Sarver, Danielle M. Schultz, Daniel A. DiRocco, and David W.C. MacMillan was supported the NIH National Institute of General Medical Sciences(R01 GM103558-03) and gifts from MSD, Bristol-Meyers Squibb, Eli Lilly, Genentech, Pfizer, and Johnson & Johnson.