Team Finds Disordered Materials with Band Gaps
Researchers from Princeton Chemistry and Princeton Physics have found a clear and reliable “winner” in the search for disordered network materials that maintain desirable optical properties even for large sample sizes.
Among a wide class of competitors, only “disordered stealthy hyperuniform” materials were found to have this feature.
The discovery advances our understanding of metamaterials that may enable scientists to replace electronic devices, such as chips for computers and communication, with more efficient photonic or light-driven devices.
The team’s research, Wave propagation and band tails of two-dimensional disordered systems in the thermodynamic limit, was published last month in the Proceedings of the National Academy of Sciences (PNAS) by Salvatore Torquato, the Lewis Bernard Professor of Natural Sciences, Professor of Chemistry and the Princeton Materials Institute; Paul Steinhardt, Princeton’s Albert Einstein Professor in Science and professor of Physics; and Michael Klatt of the Institut für Theoretische Physik II in Düsseldorf, Germany.
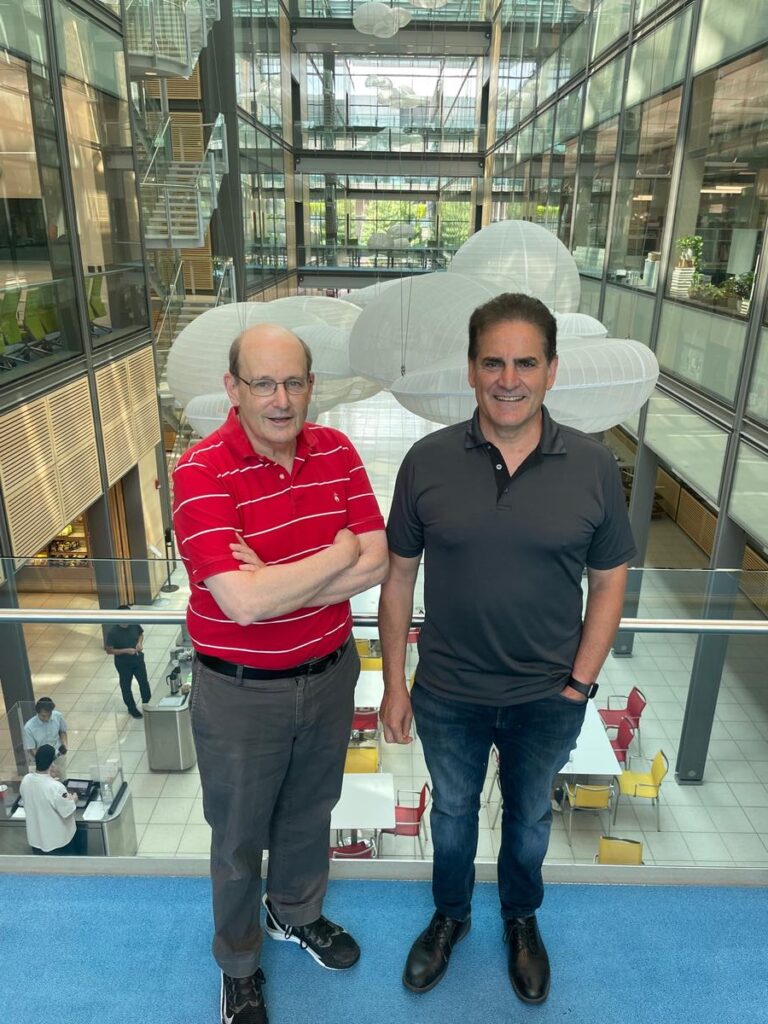
Paul Steinhardt of Princeton Physics, left, and Salvatore Torquato of Princeton Chemistry, right.
“Stealthy hyperuniform materials are an exotic disordered state that behave like crystals from intermediate to long length scales, but at short length scales are liquid-like. These are very unusual characteristics for a disordered system,” said Torquato.
“If you had a typical disordered system, you might have a little order on very short scales. Here, we’re talking about order on many length scales but yet it’s still isotropic (it appears the same in all directions). It has bizarre but beautiful characteristics that we’re just learning about. I would say we are at the infancy of understanding the nature of these stealthy hyperuniform states of matter.”
“Omnidirectional mirrors”
A key goal in photonics, or the physical science of light waves, is to develop porous materials with band gaps, meaning that the materials can block and control the flow of light for a limited range of frequencies in all directions. Such materials can be thought of as omnidirectional mirrors for certain frequencies of light that in many ways act like semiconductors—but for light rather than electrons. To design such materials, it is essential that scientists understand the relationship between the formation of photonic band gaps and the material microstructure.
Until recently, it was thought that only ordered or periodic microstructures could open substantial band gaps. Torquato and Steinhardt showed about 15 years ago that sizable band gaps could be achieved with disordered microstructures, at least for relatively small sample sizes.
But do such disordered structures still show band gaps as the sample sizes are made larger? This is an important question both practically and fundamentally.
After examining a broad range of two-dimensional isotropic networks with different degrees of correlated disorder, the team found that only stealthy hyperuniform materials could possibly possess complete photonic band gaps for both small and large sample sizes.
“Earlier studies based on measurements or simulations of relatively small samples suggested that neither stealthiness nor hyperuniformity are required for this purpose,” said Steinhardt. “In this paper, we showed the opposite is the case.
“Using a novel computational methodology, we demonstrated that band gaps close as samples get larger–no frequencies are blocked—except in the cases of sufficiently stealthy hyperuniform metamaterials.”
Proving the existence of these band gaps in materials is a computational challenge, one that was prohibitive just a decade ago because researchers could only look at small systems of a few hundred nodes, or meeting points, in a structured network. That led some researchers to accept misconceptions about “easily” achieving band gaps for a wide range of disordered systems.
While the investigation focused only on photonics, researchers predict that the findings would be the same for electronics and phononics.
“We say this because what’s underlying all of these phenomena are wave equations that are very similar to one another,” said Torquato. “So whether it’s a sound wave, an electronic wave, or a light wave, what we’ve uncovered in this work should be equally applicable, and we would be shocked if it were otherwise.”
This research was sponsored by the Army Research Office and accomplished under Cooperative Agreement Number W911NF-22-2-0103. The simulations presented in the paper were substantially performed on computational resources managed and supported by the Princeton Institute for Computational Science and Engineering (PICSciE).