Chirik/BMS Study Reinforces Utility of Cobalt for C-C Cross-Coupling
Continuing in its mission to uncover new reactivities with first-row transition metals, the Chirik Lab releases a landmark study on carbon-carbon bond formation using cobalt that underscores its utility in the synthesis of pharmaceutically relevant molecules.
The mechanistic study—one of the first of its kind for this little-understood catalyst—builds on the promise of metal complexes as more sustainable, less expensive alternatives to precious metals like palladium that have traditionally served these types of reactions.
As part of their work, researchers uncovered a rarity for metal complexes that can make C-C bonds: the formation of “high-spin” cobalt alkoxides, key intermediates along the pathway to product. They reveal this compound as the cobalt resting state during catalytic cross-coupling.
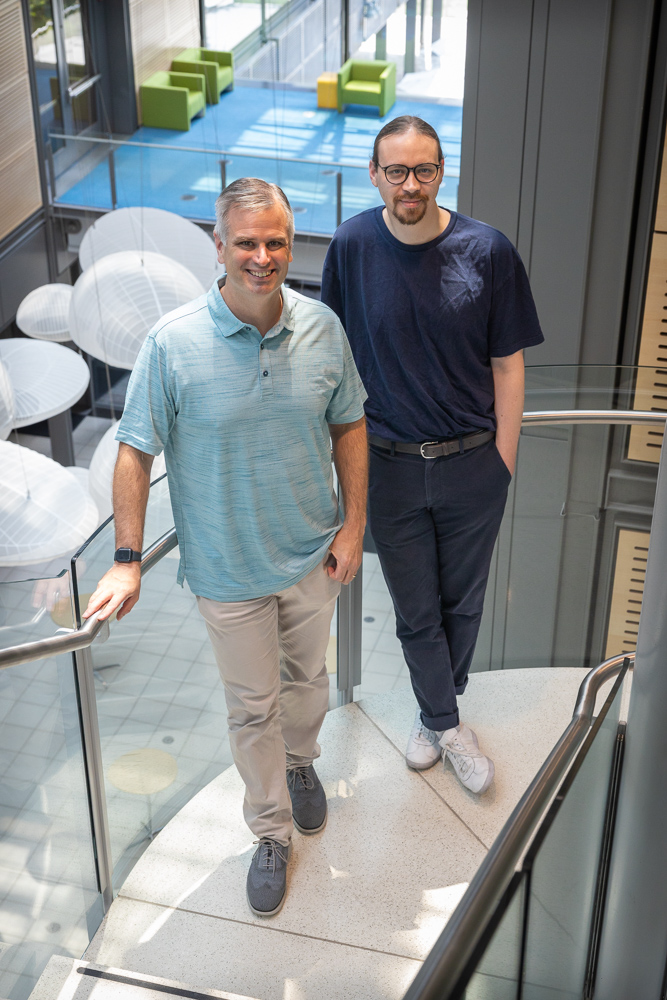
Paul Chirik (l), the Edwards S. Sanford Professor of Chemistry; and Reginald Mills, a postdoc in the Chirik Lab and the first author of the research article.
The research was a collaboration with Bristol Myers Squibb (BMS), which partnered with the Chirik Lab to uncover Earth-abundant metal catalysts for more robust, sustainable routes to C–C bonds in the synthesis of a particular drug in their portfolio.
C–C bonds are of paramount importance to synthetic chemists for the discovery and manufacture of medicines. The Chirik Lab has long had expertise in first-row metal chemistry, so BMS partnered with them to investigate whether the use of cobalt might yield a better process.
Their collaboration was funded by the Princeton Catalysis Initiative (PCI).
“Within Chemical Process Development at BMS, we have been building a platform for the development and implementation of Earth-abundant metals to facilitate transformations in our routes to clinical candidates,” said BMS collaborators Eric Simmons and Steven Wisniewski. “We anticipated this would require deep mechanistic insight into the structure and reactivity of the metal complexes involved, and the Chirik Lab is among the leading experts worldwide in the study of these metals.
“From the outset, we had very high hopes for the quality and impact of the science that would come out of our collaboration. We’re pleased to say that we’ve been thrilled to see the results continue to exceed our expectations.”
Paul Chirik, the Edwards S. Sanford Professor of Chemistry, called cobalt “a new frontier” in catalysis.
“These are new catalysts with a tremendous upside for new reactivity and ways to make carbon-carbon bonds for drug synthesis. Cobalt is a new frontier for this type of catalyst and gaining insight on how they work will allow us to make them more robust, faster and ultimately more appealing for use by BMS,” said Chirik.
“In this study, for example, we learned that the cobalt cycles between the +2 and +3 oxidation states and that cobalt alkoxides, which are pretty rare chemical entities, are key intermediates,” he said. “Knowing that cobalt alkoxides are formed in the process allows chemists to tailor new ligands and, ultimately, new catalysts to improve the reaction.”
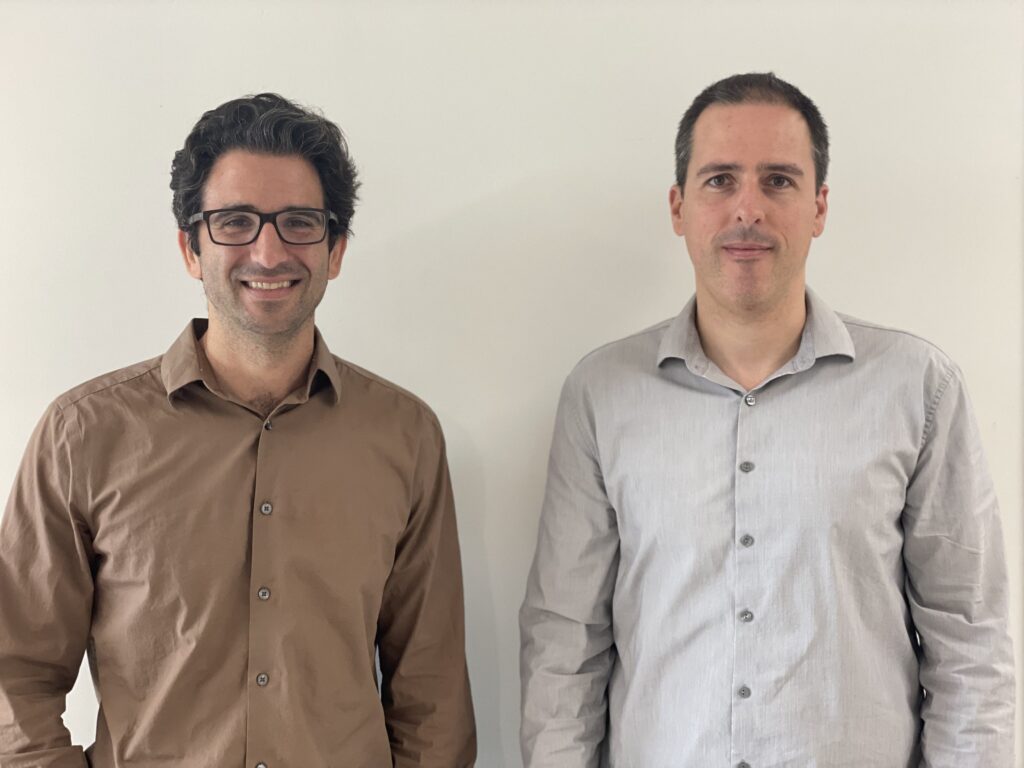
Bristol Myers Squibb collaborators in the work include Steven Wisniewski (l) and Eric Simmons (r).
The paper, Mechanistic Investigations of Phenoxyimine−Cobalt(II)-Catalyzed C(sp2)−C(sp3) Suzuki−Miyaura Cross-Coupling, was published this week in the Journal of the American Chemical Society (JACS).
How They Did It
Reginald Mills, a postdoc in the Chirik Lab and the article’s first author, said the study elucidates cobalt’s utility in ways not previously known.
“If people understand what cobalt can do, they can take a desired reaction or reactivity and say, here are maybe some of the principles of cobalt’s reactivity that we can use to design new reactions and new reactivity,” said Mills. “That’s really important. We don’t want to just do the same reactivity. We want to find new reactivity. That’s what’s exciting to chemists.”
Researchers studied Suzuki-Miyaura cross-coupling with cobalt using a combination of kinetic measurements and stoichiometric experiments. They found the catalyst particularly useful in terms of its ability to engage three-dimensional coupling partners.
“Cobalt has a unique ability to participate in chemistry with sp3 coupling partners that palladium typically doesn’t have the ability to do,” said Mills. “We’re making a bond where we still have the aryl component, but now our molecule here has a three-dimensionality to it.
“When the molecule is 3D, it often translates into better binding and better specificity to a specific target if the molecule can move in that third dimension to fit into the binding pocket.”
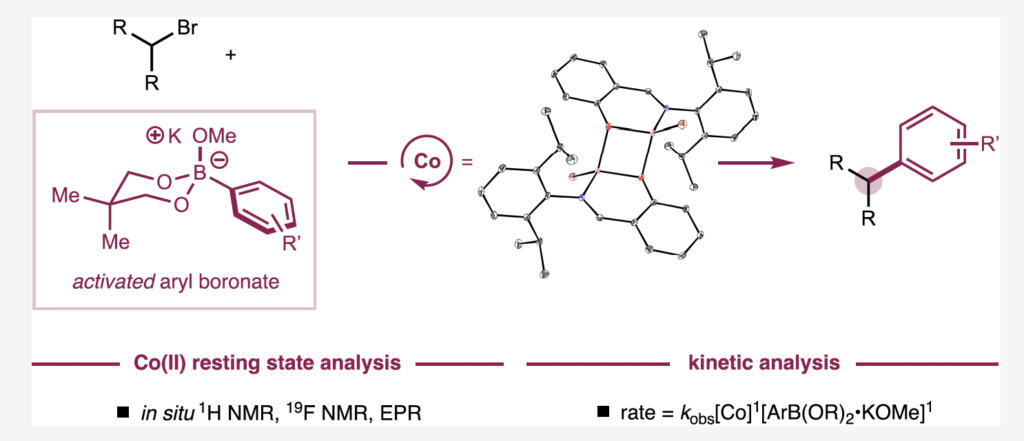
This graphic shows the cobalt resting state analysis and the kinetic analysis of this landmark study.
Many of the experiments in this study were directed to the reactivity of the cobalt resting state, or the catalyst “identity” before it performs the most challenging step in the catalytic cycle: the turnover limiting step.
“When we study the catalyst resting state, we can begin to understand that the identity and structure of the resting state is related to how the catalyst is going to do the hard stuff,” said Mills.
“One of the surprising things to us was that it’s a cobalt-alkoxide resting state, which actually performs transmetalation with the activated nucleophile to do the turnover limiting step. This was surprising because I think of an alkoxide as a bad leaving group.”
Mills also noted that researchers used a wide array of techniques to understand the cobalt catalyst, including NMR spectroscopy, electron paramagnetic resonance (EPR) spectroscopy, X-ray crystallography, and paramagnetic diffusion-ordered NMR spectroscopy.
NMR spectroscopic techniques in particular can be less diagnostic with first-row metal compounds because the unpaired electrons on the metal can interfere with resolution of the data.
“I think another of the cool things about this paper is that we figured out we can use these different methods to begin to understand what a lot of people would view as a challenging catalytic system because it’s a first-row metal system,” said Mills. “Hopefully, we’ve shown that these types of studies are feasible on first-row metals like cobalt and maybe, at some point, iron.”
This research was supported by funding from Bristol Myers Squibb through the Princeton Catalysis Initiative.