Brushing aside “dogma,” MacMillan Lab uses Marcus Theory to drive cobalt photocatalysis
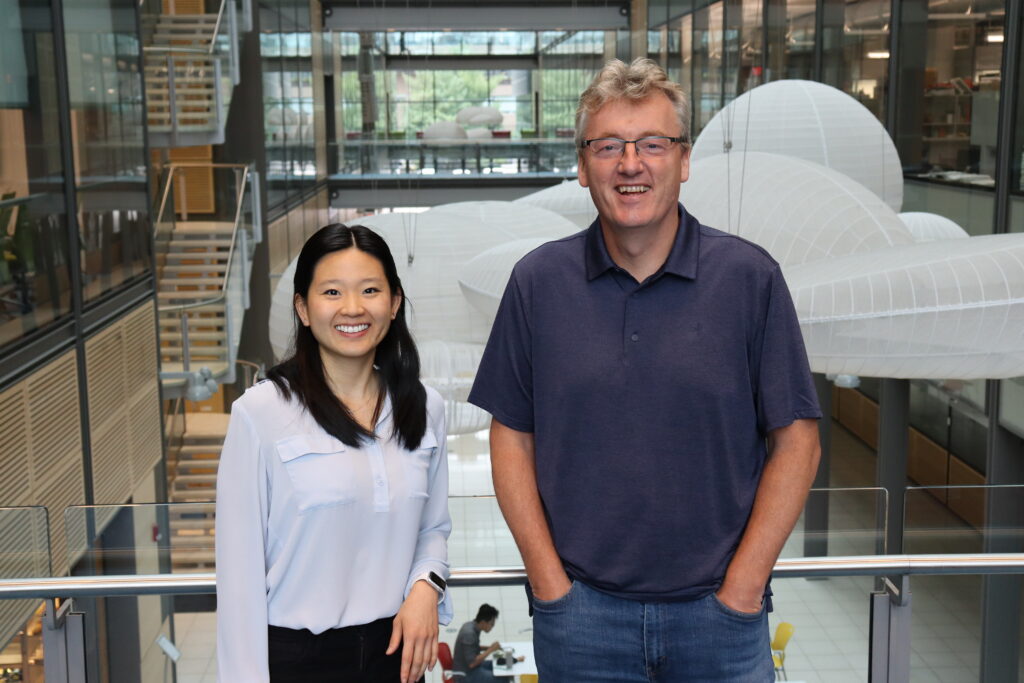
Researchers in the MacMillan Lab have unlocked a pathway to using earth-abundant metal photocatalysts with a discovery that exploits an unusual phenomenon called the Marcus inverted region.
Published in Science this month, their research enables an increase in the lifetime of excited states in cobalt complexes for straightforward, scalable bimolecular reactivity.
Traditionally, earth-abundant first-row transition metals like cobalt and iron—cheap, sustainable, plentiful elements found in the first row of the periodic table—have not been used for photocatalysis because their excited states are too short to sustain productive chemistry.
Instead, scientists have relied on second- and third-row transition metals like ruthenium and iridium. These metals have excited states that last long enough to drive chemical reactions, but they are among the rarest and most expensive on earth.
The MacMillan Lab provides an alternative that could have vast implications for designing first-row metals as photocatalysts and for unknown reactions that make use of Marcus theory.
“It was a great moment where science takes you in a direction that, given everything we know, this should not be possible,” said David MacMillan, the James S. McDonnell Distinguished University Professor of Chemistry and the co-recipient of the 2021 Nobel Prize in chemistry.
“These first-row metals were interacting with other molecules when they should not have been able to do that. We had no idea how or why. The dogma says you can’t use those metals for this. Well, the dogma’s wrong.
“I’d love to say we designed it,” MacMillan said, adding that elucidating the mechanism behind the method involved a four-year collaboration with Michigan State University. “We didn’t design it – but we did find it through some great detective work.”
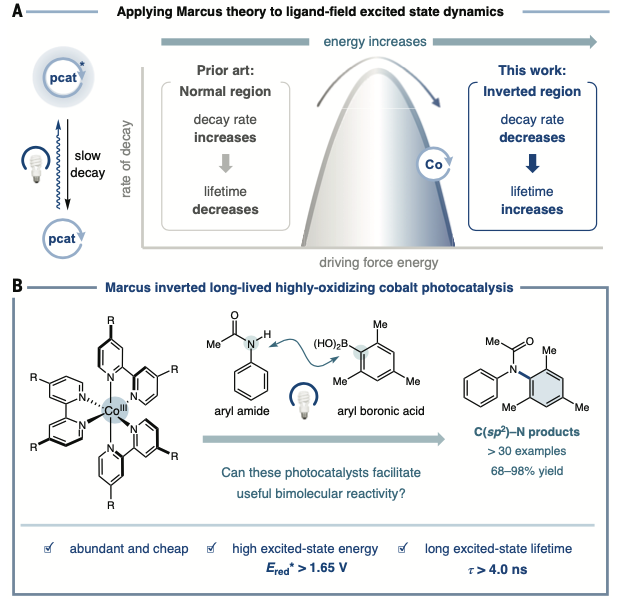
Marcus theory explains the rate by which an electron can move from one chemical species to another. While the theory is well understood, it has not previously been seen in context of extending excited states.
“Marcus theory deals with the kinetics of this electron transfer. There’s a free energy and a barrier associated with it,” said Amy Chan, first author on the research paper and a fifth-year graduate student in the lab. “The idea behind it is that as you increase the energy of this transfer from a reactant to a product state, you decrease the barrier. It becomes more favorable.
“Once you increase the energy of that so much, you’ll re-introduce a barrier, and that’s when you’re in this ‘inverted region.’ The way we’ve applied that to the excited lifetimes of these photocatalysts is to think about an excited state and its relaxation to the ground state: the more favorable the energy of that relaxation becomes, the greater we increase that driving force.
“You can push it so far that you reintroduce the barrier. And as you reintroduce the barrier, you lengthen the lifetime.”
New Barrier, New Reactivity
In photocatalysis, scientists drive chemical reactions by shining light on catalysts. Catalysts pick up energy from photons, which excite them and produce high-energy excited states. But with first-row metals, these states collapse too quickly—the energy dissipates within pico- or femtoseconds—for catalysis to take place.
Several years ago, when the lab first uncovered these longer states—long enough for bimolecular reactivity—the results captured the lab’s attention.
“Marcus inverted region is where the whole theory kind of inverts and goes in the other direction, which doesn’t make a whole lot of sense,” said MacMillan. “But Jim McCusker at Michigan and some people in my lab started to understand that maybe you can use this weird thing, this Marcus inverted region, to enable these very cheap catalysts to actually do chemistry.
“It sounded crazy. But working with Jim, we demonstrated that that’s exactly what’s going on.”
Jian Jin, a former research associate scholar in the MacMillan Lab and now a professor at the Shanghai Institute of Organic Chemistry, was one of the first to uncover the reaction at Princeton Chemistry while working with photoactive transition metal complexes.
“I wondered if we could give a shot to first-row transition metals, such as cobalt,” said Jin via email. “On an ordinary day of 2016, I mixed up a cobalt salt with a bipyridine ligand to catalyze the oxidative C–N coupling under visible light irradiation. Then joy mingled with surprise; the reaction proceeded smoothly and afforded the desired product in a high yield.
“It was demonstrated later by my talented coworkers that a cobalt photocatalyst with an unexpected but long excited-state lifetime should be there. Now I look back at the moment with undiluted pleasure.”
Chan concluded, “The key takeaway here is the design principle of increasing that driving force so much so that you can lengthen its lifetime. It’s going to open new doors to many useful transformations.”
Funding for Exploiting the Marcus inverted region for first-row transition metal-based photoredox catalysis, was supported by the NIGMS; the NIH (under Award R35GM134897); the Princeton Catalysis Initiative; BioLEC, an Energy Frontier Research Center (US Department of Energy, Office of Science, Office of Basic Energy Sciences, under Award DE-SC0019370), and gifts from Janssen, Merck, Bristol Myers Squibb, GenMab, Genentech, and Pfizer.
A.Y.C. thanks Princeton University, E. C. Taylor, and the Taylor family for an Edward C. Taylor Fellowship.